Paul Roberts Fluke Precision Measurement Ltd Norwich, UK.
Oscilloscope calibration has traditionally been considered part of the DC & LFAC workload. Nowadays the average scope found on a development engineer’s or test technician’s bench is a 1GHz bandwidth device, and bandwidths of several GHz are common. The processes and equipment used to calibrate these higher bandwidth scopes have also increased in frequency, taking oscilloscope calibration into the realm of RF & Microwave. Metrologists and calibration technicians increasingly have to deal with a variety of high frequency considerations such as VSWR and matching errors. Understanding how these sources of error in oscilloscope calibration influence the results and how oscilloscope calibration methods address them can simplify the task of scope calibration, reduce errors and ease the burden of uncertainty analysis.
Introduction
Calibrating the vertical (Y) channel accuracy of oscilloscopes is usually performed with DC or low frequency squarewave signals. Bandwidth is generally tested with a high frequency sinewaves. Nowadays bandwidths of 1GHz and 50Ohm inputs are common where previously the average scope was likely to have 250MHz bandwidth and only a high impedance (1MΩ) input. At these higher frequencies loading, impedance matching and VSWR effects can have significant impact on the measurement.
Testing the vertical channel pulse response is another measurement involving high frequency signals, in this case fast risetime pulses which contain significant high frequency content. Vertical channel risetime is usually tested by applying a high speed edge and observing the displayed transition time from 10% to 90% of the step size. Pulse aberrations (pre-shoot and overshoot) are often also measured. Impedance mis-matching between the pulse source and the scope input can cause reflections which will introduce unwanted pulse shape distortions and influence results.
The design of oscilloscope calibrators minimises the impact of these high frequency effects, but calibration technicians and metrologists must be aware of their impact, particularly in the context of laboratory accreditation and performing measurement uncertainty analyses.
Impedance matching basics
High frequency signal sources, including oscilloscope calibrators, have 50Ω outputs and are calibrated in terms of the level developed across a correctly terminated load. Errors from nominal or variation with frequency of either the source output impedance or load impedance will have an effect on the signal level developed across the load – in this case the scope input.
If a source of voltage VS and output impedance RS is connected to a load of impedance RL, the voltage developed across the load VL is given by VL = VS * RL /( RS + RL). In practice, the impedances are not purely resistive, particularly at high frequency, and the impedances are not expressed directly in terms of resistance, capacitance and inductance, but in terms of VSWR (voltage standing wave ratio) or return loss (which is related to VSWR). These parameters are high frequency terms which are used to describe how well the actual impedance varies from the nominal (50Ω) impedance. (In practice, they actually relate the incident and reflected signals at a mis-match). If either the source impedance or the load impedance is extremely close to the nominal 50Ω, the impact of difference from nominal (mis-match) of the other impedance is minimised. By careful design, the source impedance of the oscilloscope calibrator can be made very close to a perfect 50W (low VSWR). However its value should be considered when assessing the impact of the load (scope input) VSWR.
The impact of mis-match on signal level accuracy can be assessed by considering the VSWR of source and load. These parameters will usually appear in the equipment specifications.
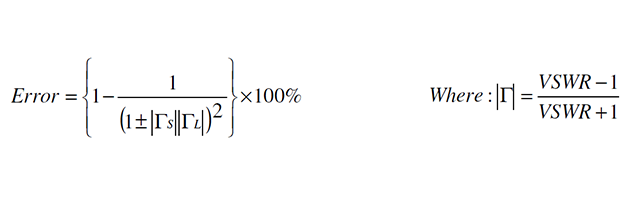
Uncertainty analysis considerations
The expression for mis-match error listed above frequently appears in RF & Microwave theory literature, deriving a value for the error in terms of power. Oscilloscopes are calibrated in terms of voltage, so the mis-match error should also be expressed in terms of voltage. If the errors are small, the power error can be halved to obtain an equivalent voltage error without significant loss in accuracy (voltage is proportional to the square root of power, and the sensitivity coefficient in the relation between voltage and power is therefore ½). Alternatively the form of the expression for mis-match error in terms of voltage can be used, as shown below.
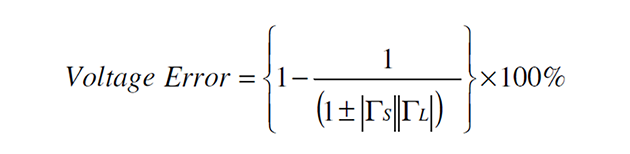
Typical oscilloscope 50Ω input VSWR can be 1.5 up to 1GHz. The higher bandwidth (4 – 6GHz) instruments are generally better achieving VSWR of 1.1 up to 2GHz, rising to 1.3 at 4 – 6GHz, with some examples exhibiting typical VSWR of 2.0 at 4GHz. On their lower (more sensitive) ranges, VSWR can be worse because there is less ‘padding’ from attenuators (which tend to improve matching) prior to the input stage. For example the Tektronix TDS6604 VSWR is specified as typically 1.3 at 6GHz for >100mV/div and 2.5 at 6GHz for <100mV/div.
Oscilloscope calibrators are designed to provide low VSWR outputs, and typical values (in this case for the Fluke 9500B with 9560 6GHz active head) are <1.1 up to 550MHz, <1.2 for 550MHz – 3GHz and <1.35 for 3Gz – 6GHz. The effect of calibrator and UUT VSWR on mis-match error is shown in the chart below, using the listed values for calibrator VSWR. The chart shows the worst case errors for particular UUT VSWR values. Note that the plots are slightly asymmetric – the amount by which a given mis-match can reduce the signal amplitude is slightly more than the amount by which it can increase the amplitude. The larger figure would usually be used as a worst case plus-or-minus contribution for uncertainty analysis purposes. (9500B users should note that the effect of UUT input VSWR of up to 1.6 is included in its published specifications).
When performing uncertainty analyses, the effect of mismatch on amplitude accuracy should be treated as one of the type B (systematic) contributions. Because of the nature of source and load mis-match errors the contribution has a U-shaped distribution (the majority of other type B contributions are treated as having rectangular or normal distributions). The uncertainty due to mis-match should be calculated from the VSWR information and divided by root two to be expressed at standard uncertainty for combination with the other uncertainty contributions.
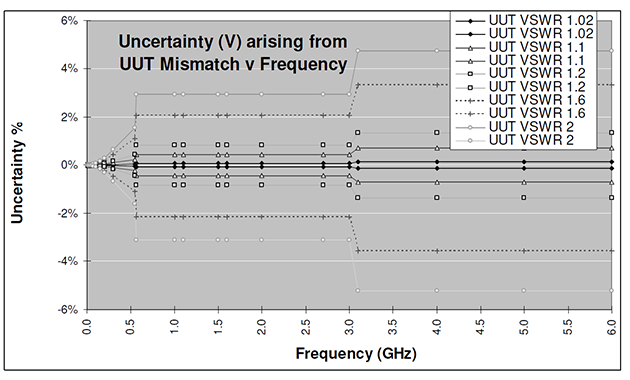
Oscilloscope bandwidth testing
The impact of mismatch on signal level accuracy can be evaluated as described above. It should then be applied to the bandwidth measurement, but the manner in which it is applied depends on the bandwidth test method.
A common technique for bandwidth testing recommended by the oscilloscope manufacturers in the verification procedures documented in their product manuals is to determine the relative display amplitude at the nominal bandwidth frequency. Usually this involves measuring the relative amount by which the displayed amplitude falls, expressed in dB, at the nominal bandwidth frequency with respect to the amplitude at a lower reference frequency and confirming the change is <3dB. The signal amplitude uncertainty can be calculated as described above and applied directly – remembering to convert the uncertainty from a linear (%) ratio to dB if the result is expressed in dB.
An alternative method is to determine the frequency at which the signal amplitude falls by 3dB relative to a lower reference frequency (to 70.7% in voltage terms). Conversion from an amplitude uncertainty to a frequency uncertainty must be performed by considering the slope of the scope frequency response. There is generally a factor of two relation between frequency and voltage uncertainty for typical oscilloscope roll-off characteristics, but this will depend on particular scope design characteristics. A simple determination can be made by deviating the frequency above and below the measured 3dB point by equal amounts (a few percent) and measuring the change in displayed amplitude. Modern oscilloscopes with readout features ease the task of determining the value of such a small amplitude change. If the roll-off is smooth without excessive ‘peaking’ close to the 3dB point the amplitude changes for equal frequency changes above and blow the 3dB point should be similar, and can be used to determine the amplitude to frequency conversion factor to be applied to the amplitude uncertainty figure.
Oscilloscope pulse response testing
The effect of mis-match on the fast edge signals used for pulse response testing will be to cause reflections. The waveform displayed on the oscilloscope will be the combined effect of the edge from the oscilloscope calibrator and the lower amplitude reflection from the scope input. The magnitude of the reflection will depend on the calibrator source and oscilloscope input VSWR, and the timing of the reflection will depend on the effective transmission line length between the source and load. The effect is minimised by the design of the scope calibrator which provides a low VSWR source, particularly for the high speed pulses available from calibrators with active head designs. Low source VSWR will ensure the majority of any reflected signal is absorbed by the source, rather than being re-reflected again.
If reflections occur they generally impact the observed pulse aberrations rather than the observed risetime because the reflections appear back at the scope input after a delay, and therefore influence a later part of the displayed pulse shape. Generally, this is the part of the waveform where the oscilloscope aberrations specifications are least stringent and therefore any reflections have minimal impact on the test outcome.
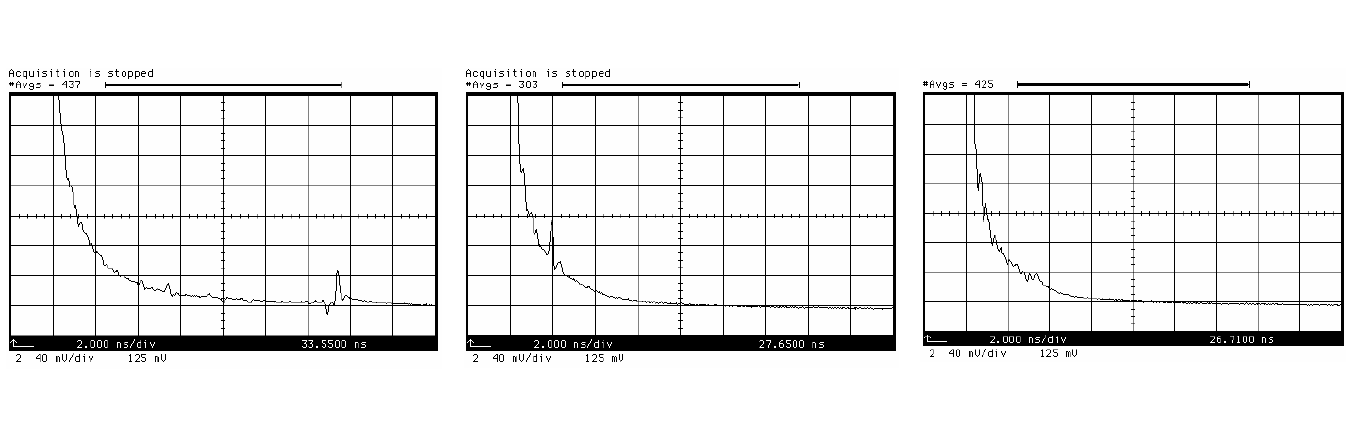
Screenshots above show effect of reflection on viewing a 150ps falling edge transition in a 20GHz bandwidth. Left: from deliberate mismatch and cable delay. Middle: mismatch with no delay. Right: absorption of reflection by good source match. Vertical scale 2%/div, horizontal scale 500ps/div.
Excessive reflections may be an indication of poor VSWR caused by damage to the scope input, for example from an accidental overload during usage.
Conclusions
The design of dedicated oscilloscope calibration solutions minimise the effect of impedance mismatches, but calibration technicians and metrologists should be aware of their impact:
- The effect of scope input VSWR can easily be assessed and included as an uncertainty contribution when making bandwidth tests.
- Mis-match effects should be considered as having a U-shaped distribution for uncertainty analysis purposes, and be treated with a coverage factor of √2 when converting to standard uncertainty.
- Mis-match effects can also influence pulse testing results by causing reflections, and excessive observed aberrations or anomalies may be indicative of scope input damage.
References
Microwave Datamate’, IFR reference data booklet, ref no 46891/861.
Fundamentals of RF and Microwave Power Measurements, Agilent Technologies, Application Note 1449-3.
Tektronix CSA7000 Series, TDS7000 Series, & TDS 6000 Series Instrument User Manual, TDS3000 Series User Manual.
Fluke 9500B Operation and Performance Handbook.
Calibration of Oscilloscopes, European cooperation for Accreditation of Laboratories, EAL-G30.